Article
Cover
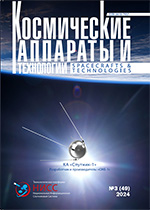
Title
Analysis of the motion of a nanosatellite with an uninsulated conductive tetherAuthors
T.A. Bystranova, Yu.M. ZabolotnovOrganization
Samara National Research University named after academician S. P. KorolevSamara, The Russian Federation
Abstract
The motion of an electrodynamic tether system consisting of a nanosatellite and an auxiliary body (electron emitter) is analyzed. The nanosatellite and the emitter are connected by an uninsulated conductive tether. The task is related to the urgent problem of removing exhausted nanosatellites or small spacecraft from space without using jet engines. The interaction of the conductive tether with the Earth's magnetic field leads to the appearance of an Ampere (Lorentz) force, which provides rapid braking of the tether system. To analyze the motion of the considered space system, a mathematical modeling method is used using equations of motion constructed using the Lagrange formalism. A feature of the mathematical model of the system motion is the consideration of the uneven current distribution along the bare conductive tether, which depends on the potential difference between the end bodies. The effect of electron concentration in the ionosphere and inclination on the current distribution along the tether and the change in the orbital parameters of the center of mass of the system is studied. It is established that for non-equatorial orbits, the motion of the tether system has a complex spatial character (it oscillates relative to the orbital plane). Numerical results are presented illustrating the effect of electron concentration and inclination on the increments of the parameters of the orbit of the center of mass of the tether system.Keywords
electrodynamic tether system, Ampere force, ionosphere, non-insulated conductive tether, nanosatellite, Lagrange equations, electron concentration, orbital inclination, current distributionReferences
[1] Zhong R., Zhu Z. H. Dynamics of Nanosatellite Deorbit by Bare Electrodynamic Tether in Low Earth Orbit // J. of Spacecraft and Rockets. 2013. Vol. 50. № 3, pp. 691–700.
[2] Beletsky V. V., Levin E. M. Dynamics of space tether systems. Moscow: Nauka, 1990. 336 p.
[3] Kulkov V. M., Egorov Yu. G., Tuzikov S. A. Investigation of the configuration and formation of the design appearance of an expanded electrodynamic cable system as part of orbital spacecraft // Izvestiya RAS. Energy. 2018. No. 3, pp. 119–130.
[4] Kulkov V. M., Egorov Yu. G., Tuzikov S. A., Firsyuk S. O. Features of construction of small-sized space electrodynamic cable systems // News of the Russian Academy of Sciences. Energy. 2019. No. 3, pp. 52–67.
[5] Ohkawa Y., Kawamoto S., Okumura T., etc. Review of KITE – Electrodynamic tether experiment on the H-II Transfer Vehicle // Acta Astronautica. 2020. Vol. 177, pp. 750–758.
[6] Chen X., Sanmartin J. R. Bare-tether cathodic contact through thermionic emission by low-work-function materials // Physics of Plasmas. 2012. Vol. 19. P. 1–8.
[7] Sanchez-Arriaga G., Bombardelli C., Chen X. Impact of Nonideal Effects on Bare Electrodynamic Tether Performance // J. of Propulsion and Power. 2015. Vol. 31(3), pp. 951–955.
[8] Liang F., Xia Q., Wang N., etc. Power Generation on a Bare Electrodynamic Tether during Debris Mitigation in Space // Int. J. of Aerospace Engineering. 2021. Art. ID 8834196. 13 p.
[9] Li G., Zhu Z. H. Parameter influence on electron collection efficiency of a bare electrodynamic tether // Science China Information Sciences. 2018. Vol. 61, Iss. 2. No. 022201.
[10] Luo C., Hao W. H., Jin D. Libration control of bare electrodynamic tether for three-dimensional deployment // Astrodynamics. 2018. Vol. 2, pp. 187–199.
[11] Zhang J., Zhu Z., Sun Z. Reduction of Libration Angle in Electrodynamic Tether Deployment by Lorentz Force // J. of Guidance Control and Dynamics. 2017. Vol. 40. No. 1, pp. 164–169.
[12] Voevodin P. S., Zabolotnov Yu. M. Modeling of the braking process of a nanosatellite using an electrodynamic cable system // Problems of control and modeling in complex systems. Proceedings of the XXI International Conference. In 2 volumes. 2019, pp. 232–237.
[13] Okhotsimsky D. E., Sikharulidze Yu. G. Fundamentals of space flight mechanics. Moscow: Nauka, 1990. 448 p.
[14] Voevodin P. S., Zabolotnov Yu. M. Analysis of dynamics and selection of parameters of an electrodynamic space cable system operating in thrust generation mode // Kosmich. research. 2020. Vol. 58. No. 1, pp. 61–72.
[15] Menon C., Kruijff M., Vavonliotis A. Design and Testing of a Space Mechanism for Tether Deployment // J. Spacecraft and Rockets. 2007. Vol. 44. No. 4, pp. 927–939.
[16] Li G., Zhu Z. H. Precise analysis of deorbiting by electrodynamic tethers using coupled multiphysics finite elements // J. of Guidance, Control, and Dynamics. 2017. Vol. 40. Iss. 12, pp. 3343–3352.
[17] Xie K., Yuan H., Liang F. and oth. Lorentz Force Characteristics of a Bare Electrodynamic Tether System with a Hollow Cathode // J. of the Astronautical Sciences. 2021. Vol. 68, pp. 327–348.
For citing this article
Bystranova T.A., Zabolotnov Yu.M. Analysis of the motion of a nanosatellite with an uninsulated conductive tether // Spacecrafts & Technologies, 2024, vol. 8, no. 3, pp. 197-206.
This Article is licensed under a Creative Commons Attribution-NonCommercial 4.0 International License (CC BY-NC 4.0).